Chemical recycling of PLA
PLA is a hard biodegradable plastic and a bio-based polymer suitable for a recycling society. It is the most researched and developed biodegradable plastic. Whether it is material recycling, heat recycling, chemical recycling, or biological recycling with biodegradation, it can be carried out. PLA has excellent hydrolysis and depolymerization properties, so chemical recycling and reuse, such as thermal decomposition, chemical hydrolysis, and hydrolysis under the action of enzymes and microorganisms, have been applied (Figure). The most important thing in the chemical recycling and reuse of PLA is to prevent the generation of isomerization.
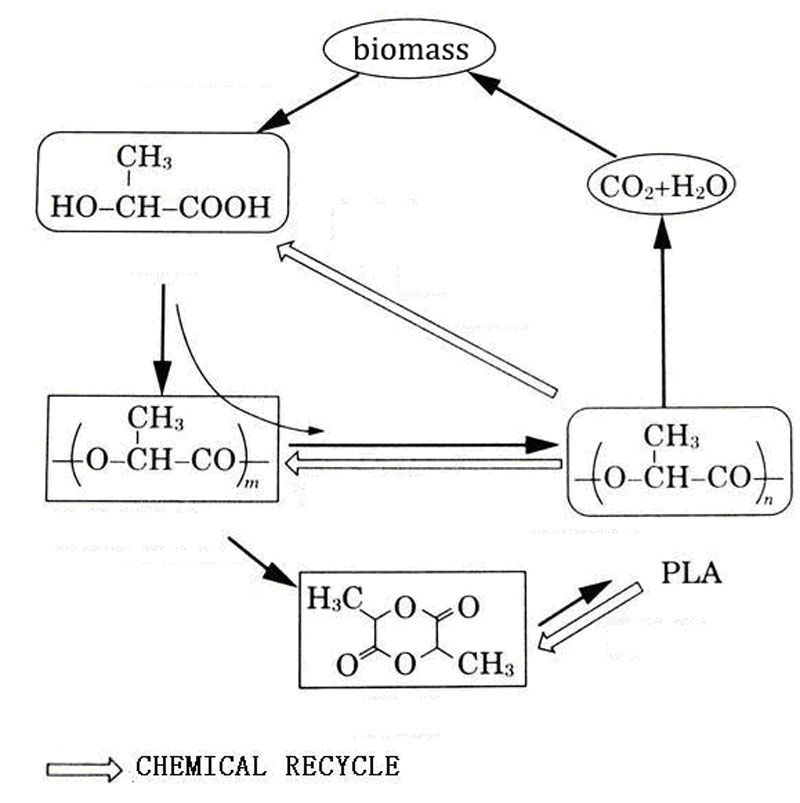
1 .Thermal decomposition method
The research on chemical recycling of PLA into cyclic lactide through thermal decomposition has a long history. In the thermal decomposition of PLA, different results can be obtained under the mutual influence of various factors. This is because the thermal decomposition mechanism of PLA is not single, but a complex combination of various mechanisms. For example, intramolecular and intermolecular transesterification, beta elimination, racemization resulting from ester-hemiacetal isomerization, and the like.
In addition, the influence of the metal catalyst residue used in the polymerization cannot be ignored. Generally, the metallization catalyst used in the polymerization is not removed, but directly remains in PLA, so it will have a significant impact on the decomposition reaction when thermally decomposed at high temperature. For example, the Sn-containing catalyst commonly used in the synthesis of lactic acid remains in the polymer, which will promote the reduction of the decomposition temperature and the selective formation of L,L-lactide. Therefore, the thermal decomposition temperature of metal-free refined PLA is higher than that of unrefined PLA, so it is easier to produce alcoholysis and isomerization reactions at high temperatures. If alkaline earth metals such as calcium and magnesium are added at this time, the decomposition reaction of PLA can be controlled to selectively generate L,L-lactide at a specific temperature. In addition, by using a suitable catalyst, the decomposition temperature of PLA can be reduced to below 100°C. In this way, even if it is mixed with general-purpose resins such as PE and PS whose thermal decomposition temperature is higher than 350°C, PLA can be decomposed only into lactide. Selective recycling.
In addition, the technology of decomposing PLA into lactide using supercritical carbon dioxide with both liquid and gas properties is also under research and development. Generally, the reaction conditions are 100°C, high temperature and high pressure of 100-200 atm, plus organic solvent and catalyst.
2 Water splitting method
PLA is more prone to hydrolysis under alkaline conditions. For example, PLA particles can be completely decomposed into lactic acid monomers after being stirred in 10% ammonia water at 80 °C for 2 hours. Moreover, by this method, only PLA can be selectively decomposed into lactic acid monomers from the PLA-ethylene polymer mixture.
There is also a decomposition method that does not use a water-soluble acid-base catalyst, but uses a solid acid. Solid acids are insoluble in solvents and are easier to separate. The solid acid can be separated and reused by filtration. Therefore, the chemical recycling method of decomposing PLA into oligomers and monomers using a solid acid catalyst is more environmentally friendly than the previous thermal decomposition and the method using acid and base catalysis. As can be seen from Figure, the production of PLA from lactic acid, whether it is a direct polycondensation route or a route via lactide, will generate an intermediate lactic acid oligomer. Therefore, in the chemical recycling and reuse of PLA, from the perspective of energy It is more advantageous to fall back to lactic acid oligomers for use as low-molecular raw materials.
Kaolin, a low-cost and environmentally friendly clay mineral that can be used as a solid acid catalyst, is very effective for the decomposition of PLA. This is determined by the layer structure of kaolin, the carbonyl group of PLA is adsorbed to the interlayer part of kaolin, and under the interaction there, the carbonyl group is activated and is more susceptible to nucleophilic attack by the water present in the interlayer. This results in a reaction similar to that occurring at the active site in an enzymatic reaction, accelerating the progress of decomposition.
For example, in Figure, the transparent cup made of PLA used in the Aichi Expo in Japan is chemically recycled and reused under the action of kaolin. PLA with average molecular weight Mw=120,000 was dissolved in toluene at 100°C, kaolin was added, and after stirring for 1 hour at constant temperature, PLA was decomposed into lactic acid and lactic acid oligomers with average molecular weight Mw=250. During this sequence, almost no D-isomer of lactate occurs. The resulting monomer/oligomer mixture is easier to polymerize than previous chemically catalyzed mixtures to regenerate PLA. That is, using an oligomer with an average molecular weight Mw=250, and performing solid-phase polymerization under the catalysis of SnCl2/p-TSA, PLA with an average molecular weight Mw=170,000 can be obtained. The glass transition temperature, crystallization temperature, and melting point of the obtained PLA were basically unchanged from those of the original raw materials. Moreover, the kaolin catalyst can be reused after a simple recovery process.
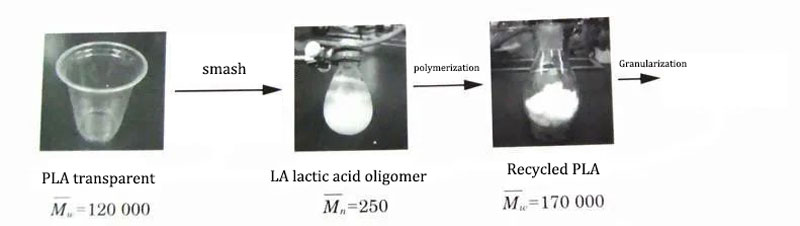
3.Chemical recycling of PHA
PHA is one of the representative bio-based polymers, and is regarded as a potential bio-based polymer like PLA. Figures are diagrams of the synthesis and chemical recycling of bio-based polymers. P(3HB) is cleaved to 3HB by P(3HB) depolymerase.
However, due to the molecular structure of PHA, chemical decomposition using commonly used heat or acid and alkaline catalysts will eliminate hydroxyl groups on the polymer end groups to generate crotonate functional groups, and finally generate free crotonic acid through liquid phase depolymerization. Therefore, it is difficult to selectively obtain oxoacid monomers (Figure ). The chemical recycling method of converting P(3HB) into crotonic acid by thermal decomposition has also been tried, and the activation energy of the reaction is Ea=110~380kJ/mol. However, since a unified numerical value cannot be obtained, further research and discussion on various factors including the decomposition mechanism are required.

Enzymatic catalysis can also be used to recycle biopolymers through chemical recycling to produce repolymerizable feedstocks. The first step in the biodegradation of solid state PHA is hydrolysis under the action of P(3HB) depolymerase until the oligomers are decomposed into monomers. On the other hand, in organic solvents, PHA can be quantitatively ring-opened and polymerized into cyclic oligomers under the action of lipase. For example, a toluene solution of PHA can be substantially quantitatively converted into cyclic oligomers under the action of immobilized lipase B. The obtained cyclic oligomer can generate PHA again by its own ring-opening polymerization (Fig). Furthermore, since this cyclic oligomer can be polymerized with various lactones, it is possible to design various polymers with physical properties that are not found in the original bio-based polymers derived from microorganisms, and can also be regarded as advanced recycling. Similarly, unnatural P(R,S-3HB) can also be cyclic oligomerized.